An Introduction to Pulsars
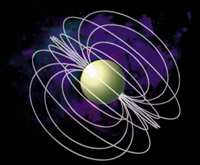
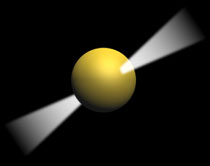
This webpage has been designed to give you an understanding of pulsar astronomy. We start by describing how astronomers discover and subsequently observe pulsars. We continue with a section on the theory of pulsars where we explain why pulsars are thought to be neutron stars (the collapsed core of a star that underwent a supernova explosion). We explain how these pulsars are actually studied today and what we can learn from these amazing objects. We finish by providing links to other webpages for those of you who would like to read more about pulsars.
Observations of Pulsars
The first pulsar was discovered by chance by Jocelyn Bell and Anthony Hewish in 1967 who were actually studying distant galaxies at the time. Jocelyn Bell noticed small pulses of radiation when their telescope was looking at a particular position in the sky and for a short time scientists thought they might be coming from an extra-terrestrial civilisation. In fact the source of these pulses were initially referred to as LGM1, Little Green Man 1. Once established that the signals were not of this origin (and also not caused by people on Earth), the unidentified object they were coming from was called a "pulsar" because the emission was pulsed. The pulsar discovered by Bell and Hewish is now called PSR B1919+21: PSR stands for Pulsating Source of Radio and B1919+21 indicates the position of the pulsar in the sky. Even though pulsars were first discovered as radio sources they have now been observed using optical, X-ray and gamma-ray telescopes.
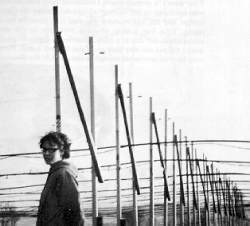
Pulsar astronomers have now detected over 1500 pulsars and expect to discover thousands more during the next few years. More than two-thirds of the currently known pulsars were discovered using the Parkes radio telescope (the star of the film "The Dish"). The enormous Arecibo radio telescope in Puerto Rico, the Green Bank telescopes in America, the Molonglo telescope in Australia and the Jodrell Bank telescope in England have also made significant contributions in discovering pulsars.
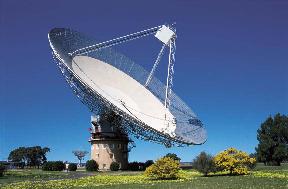
An astronomer who is searching for a pulsar will position the radio telescope at an area of sky for between a few minutes and twelve hours (longer observations allow the astronomer to detect weaker pulsars, but it requires the drinking of lots of strong coffee to stay awake!). The output of the telescope is continuously recorded by a computer that needs plenty of storage space - a recent survey at Parkes stored the equivalent of 8000 CDs worth of data. Later, the data are searched for periodic signals of the type found by Jocelyn Bell which, if found, confirm the presence of a pulsar. If the telescope was plugged into a suitable loud-speaker (instead of recording the data on to a computer system) and a bright enough pulsar was being observed then you would actually hear the signal.
No wonder they were first thought to be extra-terrestrials trying to communicate with us! (More sounds of pulsars are available at the Jodrell Bank pulsar webpage)
The time interval between consecutive pulses is called the pulsar's period. Periods of one second are typical although pulsars have been discovered with periods from a few milliseconds (one millisecond equals 0.001 seconds) up to eight seconds. The time between pulses is incredibly regular and can be measured very precisely. For example, a pulsar called PSR J1603-7202 is known to have a period of 0.0148419520154668 seconds. However the periods of all radio pulsars are increasing extremely slowly. The period of PSR J1603-7202 increases by just 0.0000005 seconds every million years! There are two main types of pulsar. Those with periods of a few milliseconds and whose periods are changing very slowly are called the millisecond pulsars. The remainder are simply called the "ordinary pulsars".
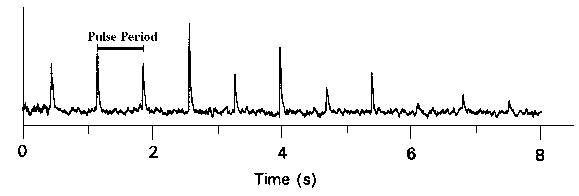
Before explaining what pulsars actually are let us consider what observations of pulsars tell us. Each pulse is found to be made up of radio waves of different frequencies just as white light is made up of all the colours of the spectrum. It is observed that the highest frequencies of a pulse arrive at a telescope slightly before the lower frequencies. The reason for this is that the pulse has been travelling through the interstellar medium (the space between the pulsar and the Earth) and the different frequencies making up the pulse travel at different speeds through this medium. This is referred to as the pulse dispersion and is due to the free electrons in the interstellar medium. The more distant pulsars are dispersed more than the closer ones and so the time delays between the different frequencies can be used to estimate an approximate distance to a pulsar. Except for a few pulsars in our neighbouring galaxies, the Magellanic Clouds, most pulsars are found to be well outside of our solar system but within our Galaxy. The youngest pulsars (we call them young, but these pulsars are many thousands of years old) are found to lie within the plane of our Milky Way Galaxy. The very youngest are found within supernova remnants which suggests that they were probably "born" during the explosion of a massive star.
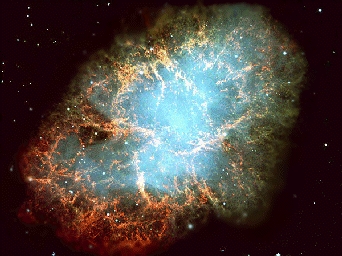
These young pulsars are found to be travelling through space very fast. In fact some pulsars are moving 4000 times faster than a jumbo jet and would take only 16 seconds to travel between Sydney and London! As they age they move away from the plane of the Galaxy. The fastest pulsars will never come back - they will escape from the Galaxy and will travel off into the space between galaxies becoming undetectable. Others will slow down and then drop back towards the plane of the Galaxy and will continue to oscillate up and down for the rest of their lives.
Most stars in our Galaxy are in an orbit with another star (our Sun is unusual in that it has no stellar companion). Similarly, many pulsars (in particular the millisecond pulsars) are found in binary systems. The companions to pulsars have been found to be normal stars, planets, white dwarf stars, neutron stars and even, for one recent discovery, another pulsar. Studying the pulsar's motion in a binary system allows astronomers to determine many facts about the pulsar, its companion and the orbit. For some systems, the mass of the pulsar can be determined and is found to be roughly one and a half times as massive as our Sun. We also know that pulsars are very small and so they must be very dense. In fact, one teaspoon of pulsar material would weigh a billion tonnes if we brought it to the surface of the Earth.
So what have we learnt? The observations of pulsars tell us that:
- they are far away
- they are very small
- they are very dense
- they can orbit other objects
- they were probably born in supernova explosions
- they are travelling very fast through space
- the time between pulses is extremely regular although they are slowing down very slightly.
In the next section we attempt to put all these observational results together to form a picture of what a pulsar actually is.
Theory of Pulsars
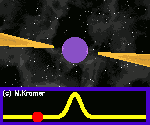
In 1934 Walter Baade and Fritz Zwicky predicted the existence of neutron stars: stars which have collapsed under their own gravity during a supernova explosion. Stars like our Sun will not form neutron stars. After exhausting all their fuel, such small stars become white dwarfs. Only very massive stars (at least a few times more massive than our Sun) will undergo a supernova explosion and become neutron stars. Even more massive stars will collapse to form black holes.
It was thought that neutron stars would never be detectable using telescopes on Earth. They were predicted to be very dense, to spin very fast, have a tiny radius of only about 10km and to possess large magnetic fields. However, we now know that charged particles moving along the magnetic field could cause beams of radiation to be emitted from the magnetic poles. Then, as the neutron star rotates, the beam would sweep across space. When this beam is in the direction of the Earth, a pulse may be detectable using a radio telescope (see the animation above). Could this "lighthouse model" answer the question of what a pulsar is?
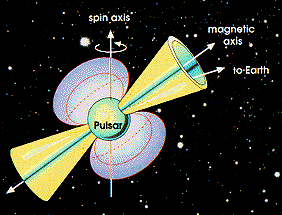
If we compare the observations of pulsars mentioned in the first section with the description of neutron stars in the second we find many similarities. The pulses that occur at regular intervals correspond to a beam being emitted from a rotating neutron star. The time between pulses, the period, is the time that it takes for the neutron star to rotate once. The increase in the period is due to the pulsar slowing down slightly as it loses energy. The youngest pulsars are found in supernova remnants which is exactly the place we'd expect neutron stars to be born. Therefore the most likely explanation is that a pulsar is a neutron star that spins rapidly and emits radio waves along its magnetic axis. However, not all neutron stars are necessarily detectable as pulsars. The beams from some neutron stars may never pass the Earth and will therefore not be detected. Also, other neutron stars may have been pulsars in the past, but the process that causes the beam of radiation (which is not fully understood) may have turned off or is just too weak to be detected.
The types of pulsar - the ordinary and millisecond pulsars - can be explained by assuming that all of the millisecond pulsars were originally in orbit with another star. After the pulsar formed, matter was pulled from the companion star on to the pulsar. During this process the pulsar rotated faster and faster until it became one of the millisecond pulsars. Later, the companion star died and became either a white dwarf, neutron star or black hole depending on its original size. If the companion star remained in orbit with the pulsar, a binary millisecond pulsar system would be formed.
Nobody is quite sure of what exactly happens to a pulsar as it ages and slows down. It is likely that after a few million years the beam of radiation switches off. This may be because the pulsar's magnetic field decays away or that the beam just gets weaker and weaker until it is undetectable. Such problems may be solved by studying pulsars discovered in recent surveys.
Current Research
The Parkes multibeam pulsar surveys
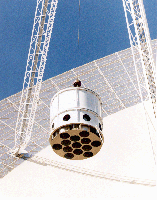
In January 1997 a 13-beam receiver was installed on the Parkes radio telescope. This receiver, the multibeam receiver, was initially built to search for galaxies but has been used by many astronomers in their search for pulsars. In an investigation that has just been completed, pulsar astronomers from all over the world have collaborated in a search for pulsars in the plane of our Galaxy and have discovered more than 700 pulsars. This phenomenal success was mainly due to the receiver that allowed 13 areas of the sky to be observed simultaneously. Because of this, the astronomers could afford to spend much longer on any particular area of sky than was possible in the past. Such long observations allowed the survey to detect weaker pulsar signals than those found during previous surveys. Quite a few of the new discoveries are interesting and include young pulsars, distant pulsars, pulsars in binary systems and even more millisecond pulsars.
Other pulsar surveys have also been carried out using this receiver. One, the high latitude survey, was mainly a search for millisecond pulsars. This survey did not discover as many pulsars as the survey we described above, but it found what is probably the most exciting pulsar system known. We'll discuss this discovery in the next section.
The double pulsar system
Astronomers using the Parkes radio telescope have discovered a pulsar in orbit with another pulsar. This has caused great excitement in the astronomical community because these pulsars allow theories of gravity to be tested with phenomenal accuracy. We already know that millisecond pulsars in binary systems are superb probes for studying Einstein's general theory of relativity. The orbits of such pulsar systems have been studied for many years to see if the observations agree with prediction. Einstein's theory agrees perfectly with the observations. The new double pulsar system will allow even better tests to be made.
Timing array projects
Many astronomers around the world work together to make regular observations of a few millisecond pulsars. These observations can subsequently be used to investigate how stable atomic clocks on Earth are, to improve our understanding of the masses and motions of the planets in our solar system and, with luck, to detect gravity waves. Gravity waves are ripples in the fabric of space and time and come from very extreme regions of spacetime such as the very early Universe and the cores of galaxies. Therefore, by studying a pulsar we can improve clocks on Earth and, at the same time, study the very early Universe and colliding supermassive black holes at the centres of galaxies!
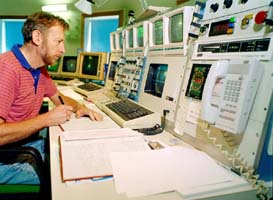
The interior structure of neutron stars
Neutron stars are only the size of a city and are very far away yet astronomers can work out what is going on beneath their surfaces - how? They do this by looking for small changes in the rotation of the pulsar. We have already mentioned that pulsars slow down as they get older. However, for a few pulsars they suddenly start spinning faster (in a very short space of time) and then slowly return to their original spin rate; that is they glitch. The Crab pulsar which is in the Crab nebula (shown in a picture above) has been observed to do this many times. The reason for glitching is not very well understood and is actively being investigated. However, current explanations suggest that glitches are probably due to the way the interior superfluid of a neutron star interacts with the crystalline surface.
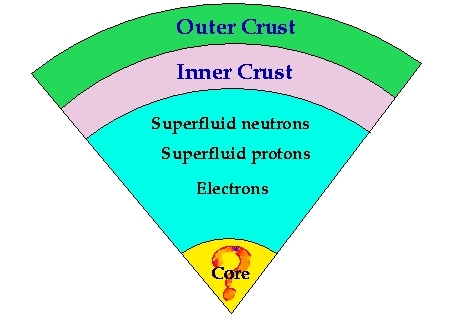
The SKA (Square Kilometre Array)
The SKA will be one of the next generation radio telescopes. It will have far greater sensitivities than any current radio telescope because its large total collecting area will be one square kilometre. This will allow much fainter pulsars to be seen. It will probably not be a single surface but will consist of many smaller collecting antennas. Astronomers are hoping that this new telescope will allow them to discover a pulsar orbiting a black hole. If found, this will be the most extreme pulsar system known. It will enable more general relativity tests to be done and alternative theories of gravity to be investigated. The SKA will also enable astronomers to find pulsars in distant galaxies.
Want to Learn More?
There are many sites on the web that contain information about pulsars. Here are a few that we find particularly interesting.
The ATNF pulsar catalogue provides information on all the known radio pulsars.
Cosmic Search Magazine contains an article by Jocelyn Bell giving an account of the discovery of pulsars.
Cambridge Physics - Discovery of Pulsars provides another introduction to pulsar astronomy.
Pulsars at Arecibo describes the research carried out at the Arecibo Observatory.
Pulsars at Jodrell Bank Observatory provides more advanced tutorials on pulsar astronomy.