Next-generation Radio Telescopes
Note added November 2016: This page dates from around 2010 and some of the information on timescales etc is now well out of date. However, the sections on SKA science remain largely correct, and so this page will be left as-is for now and will be updated in the near future.
In the 1990s astronomers took big steps in investigating the cosmic microwave background – radiation showing the state of the Universe just a few hundred thousand years after the Big Bang. The slight irregularities in this radiation are the ‘seeds’ from which the present day galaxies grew. In the same decade, the Hubble Space Telescope observed galaxies that existed when the Universe was less than a billion years old, about 6% of its current age. And the new, large, ground-based optical telescopes, such as Keck, the Very Large Telescope and Gemini, began to detect significant numbers of distant (high redshift) galaxies.
But we have not yet seen the first stars and galaxies that formed after the Big Bang. This is now one of the major goals of astronomy. So too are tracing how galaxies have evolved from their first formation until the present, and learning more about the formation of stars and planets.
To pursue these questions, radio astronomers are moving to using both very short (millimetre) radio wavelengths, and long ones (up to a couple of metres in length).
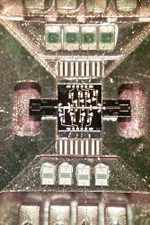
Millimetre-wave observing
The radiation from extremely distant galaxies is redshifted, "stretched" from its original wavelengths, into the longer wavelengths of the infrared, submillimetre and even millimetre-wave bands of the electromagnetic spectrum. To study it–and more nearby phenomena, such as outflows from young stars and gravitational lenses–astronomers around the world are planning specific new radio telescopes. The USA and Europe are building ALMA, a giant millimetre-wave array with some tens of dishes on the Atacama plateau in Chile.
Over the last few years the Australia Telescope Compact Array has been upgraded, under the Commonwealth Government’s Major National Research Facilities program, to work at millimetre wavelengths. This will make the Compact Array uniquely able to do millimetre-wave imaging from the Southern Hemisphere. The Compact Array will have more collecting area than any other array in the world (this will be very valuable, especially for work on highly redshifted molecular lines), and will be the world’s the world's most powerful array at 3-mm wavelengths until ALMA starts operating. (The first ALMA observations are currently scheduled for 2007, although the telescope array will be under construction until 2012.)
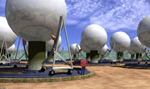
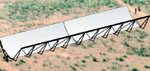

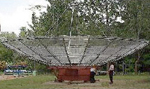
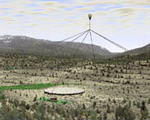
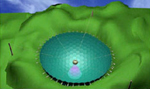
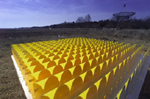
- an array of 7-m Luneburg lenses. (Image: Chris Fluke, Swinburne University of Technology, Centre for Astrophysics and Supercomputing);
- an array of parabolic cylindrical reflectors;
- small, steerable reflector antennas, like those of the Allen Telescope Array shown here. (Credit: I. Gary);
- small mesh parabolic reflectors;
- a set of large, low-profile parabolic reflectors of very long focal length;
- a set of large spherical reflectors;
- a planar array with no moving parts, steered electronically.
The Square Kilometre Array
- www.skatelescope.org - international website
- www.ska.gov.au - the Australian SKA coordination committee (ASCC)
Australia is one of seventeen countries planning for the ‘next generation’ radio telescope, which will make use of long radio wavelengths. This is the Square Kilometre Array (SKA): a telescope with a total collecting area of one square kilometre (1 million square metres). This collecting area will give the SKA about 100 times the sensitivity (ability to detect weak signals) of today’s best radio ‘interferometer’ telescopes (telescopes like the Australia Telescope Compact Array). The collecting area will not be a single surface, but made up of many smaller collecting elements: ‘antennas’.
Timing
A site for the SKA will be chosen in 2011-12, and construction of phase one is scheduled for 2013-2020.
Who will pay for and use the SKA?
The total cost of the telescope is expected to be about 1 billion Euros and will be shared among the participating countries: currently, these are Australia, Argentina, Canada, China, France, Germany, Italy, the Netherlands, India, New Zealand, Poland, Russia, Spain, Sweden, the UK, South Africa and the USA. Several countries are researching the design of the telescope. When the SKA is built it will be used by astronomers from all over the world, including Australia, regardless of where it is located.
Design
The favoured design for the SKA has as its basic "building block" a close-packed group of small antennas covering an area 30-300 metres in diameter. Such a group is called an ‘array-station’.
The whole SKA will be made up of many sparsely scattered array-stations, perhaps as many as 1000: the number will depend on the conclusions of design studies currently under way. About half the array-stations will be clustered together in a central site of about 10 km in diameter. The others will be spread over a larger distance, perhaps as much as 2 000 km from the central site.
The design of the individual antennas has not yet been finalised. Proposals have ranged from parabolic and cylindrical reflectors to spherical Luneburg lenses and small flat elements. No single concept meets all the instrument’s technical goals as they are now formulated, and so hybrid concepts are now being explored. The next step in assessing the various concepts is the building of demonstration systems. The design concept will be chosen in 2008.
CSIRO SKA research
Research on SKA science and engineering in Australia is led by CSIRO and covers a number of areas:
- Antenna elements, phased arrays and beamforming
- Energy systems for the telescope
- Integrated RF Systems
- Signal Encoding and Transmission
- Signal Processing
- Demonstrator systems
- Site testing and investigation
- Simulations of the SKA’s operations.
CSIRO is an important member of the ASCC's "Team Australia", which is helping to coordinate Australia's SKA bid. As part of this bid CSIRO is building a pathfinder instrument, the Australian SKA Pathfinder (ASKAP), which will trial novel receiver technology and demonstrate Australia's capability to host the full SKA.
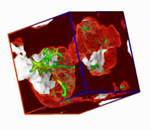
Credit: Nick Gnedin.
Quicktime movies
Major areas of science with the SKA
The first stars, galaxies and quasars
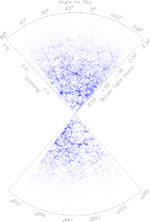
The clustering of galaxies in the (relatively) nearby Universe
SKA measurements of galaxy clustering at much earlier times in the Universe's history will be important in determining the 'equation of state' of Dark Energy. Image: 2dF Galaxy Redshift Survey
Immediately after the Big Bang, the Universe was extremely hot, and awash with light and other electromagnetic radiation. As the Universe expanded, it cooled. About 370 000 years after the Big Bang it had cooled enough for the electrons and protons to unite, forming neutral hydrogen atoms. The Universe was now dark, because no stars had formed yet. Even if light had been present, it wouldn’t have been able to travel through the Universe, as the hydrogen formed a thick, opaque fog.
As the Universe cooled further, its hydrogen gas began to form clumps, which eventually developed into galaxies, stars and quasars. Ultraviolet light from the first stars and quasars reionised the hydrogen gas, making the Universe transparent to light.
The SKA will be able to map the distribution of neutral hydrogen gas at distances beyond the reach of current radio telescopes, by detecting the radio waves it absorbs and emits. Astronomers hope to be able to detect the hydrogen gas that existed before and during the time that the first stars and quasars formed, helping us to understand the formation process.
Like other radiation travelling through space, the radio waves emitted by hydrogen gas are redshifted (stretched in wavelength) by the expanding Universe. As a result, waves reaching us from more distant parts of the Universe–earlier times–are longer than waves emitted by more nearby material. And so, by making a series of observations at different redshifts (corresponding to different times in the Universe’s history), the SKA will be able to trace how the hydrogen gas clumps grew over time.
Dark Energy
In 1998, two groups of astronomers studying supernovae (exploding stars) in distant galaxies announced that they had evidence that the Universe was not just expanding, but expanding at an ever-increasing rate: the expansion was accelerating. Later evidence, particularly from the WMAP observations of the cosmic microwave background, has supported this finding. The cause of the expansion was dubbed “dark energy”. And it appears that some 70-75% of the energy/matter in the Universe is in this form. (Only about 4% exists as familiar “baryonic” matter; a further 20-25% is non-baryonic “dark matter”, itself still mysterious.)
Astronomers describe the relationship between the outward pressure exerted by the dark energy, and its density (i.e. how much dark energy there is per unit volume of space), with an “equation of state” for dark energy. Determining the equation of state for dark matter, and how it may have changed over time, is now one of the big tasks in cosmology. An important way to measure it for early epochs of the Universe’s history is to determine how highly clustered galaxies were at that time. The SKA will be able to make the accurate measurements of high-redshift galaxies needed to do this.
Testing theories of gravity
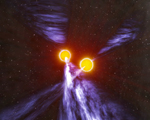
Credit: John Rowe
To date, the general theory of relativity–Einstein's theory of gravity–has passed all the astrophysical tests that have been applied to it. But there are other, alternative, theories of gravity. In particular, physicists are striving to combine the insights gained from relativity and quantum mechanics in a theory of “quantum gravity”. Some of these alternative theories predict effects very close to those of general relativity in “weak field” conditions–in the solar system, for instance–but quite different effects when applied to compact bodies such as neutron stars.
Radio pulsars are neutron stars that emit beams of radio waves, beams that we detect as pulses as the star spins and its beam sweeps over the Earth’s surface. They are extremely accurate clocks. Pulsars have been used for tests of general relativity, tests that it has so far passed. But the tests carried out so far are only a small number of the tests possible: in principle one can do up to 15 on a binary pulsar system, if the pulses are measured precisely enough. To test general relativity and other theories of gravity to the utmost, astronomers want to find a pulsar orbiting a black hole–the most extreme pulsar system possible. The SKA will be able to measure these properties and so provide the ultimate astrophysical test of theories of gravity.
A gravity wave passing through spacetime would cause the pulses emitted by a pulsar to change a little in the time they were detected on Earth. The SKA will be able to time a number of pulsars to accuracies of better than a microsecond, and so will be able to detect gravity waves, the ripples in the overall geometry of space produced by moving objects.The Universe is predicted to be awash with gravity waves that originated with the Big Bang. The SKA would be uniquely able to detect such waves, by accurately tracking the arrival times of pulses from a large number of pulsars.
Mapping magnetic fields
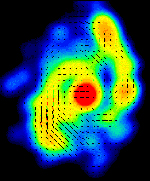
Observers: M.Ehle, R.Beck, R.Haynes
Magnetic fields pervade the Universe, but their origins and effects are little understood. Is the overall Universe magnetic? How do magnetic fields affect how stars and galaxies form and evolve? Why do galaxies have magnetic fields?
Magnetic fields can traced from radio emission: when polarised radio waves encounter a magnetic field, the plane of polarisation is rotated. (This phenomenon, known as Faraday rotation, was first discovered with the Parkes radio telescope.) The plane of polarisation can be detected and used to map the direction and strength of the magnetic field. In this way we can already map the magnetic fields of galaxies, for example. But if the SKA is pointed anywhere in the sky, it will detect the radio emission from thousands of distant galaxies. These galaxies will appear so closely spaced on the sky that we will be able to use their polarised radio emission to make detailed studies of the magnetic field throughout the Universe, perhaps even in ‘empty’ intergalactic space.
Observing planets forming around other stars

Planets form in the dusty disks surrounding young stars. This dusty material absorbs light from the parent star and re-emits it at radio wavelengths, wavelengths the SKA will be able to detect. The SKA may be the only instrument that can image the inner regions of the disks, where Earth-like planets are likely to be located. It will have the resolving power to identify features in the disks related to planet formation, such as gaps and spiral waves; to follow how these features evolve; and to identify rapid gravitational instabilities.
The SKA might also be able to detect radio transmissions that would provide evidence of technological civilisations around other stars. It will be sensitive enough to be able to detect signals as strong as those from television transmitters, if these existed on planets around the closest stars to the Sun. The SKA will be able to make the first regular searches for signals that are “leaking” off planets rather than deliberately broadcast.
Discovering the unexpected
Scientists have predicted the experiments they will be able to perform for the first time with the SKA. They believe they will solve many current mysteries with the new telescope. However, history shows that innovative new equipment such as the SKA makes unforeseen discoveries that radically change our view of nature. We expect the SKA to fundamentally change our understanding of the universe.
Location

Photo: CSIRO
Australia is one of two countries short-listed to host the SKA, South Africa being the other. CSIRO Australia Telescope National Facility and State Governments are investigating possible locations in inland Australia.
Why would Australia be a good host country for the SKA? An important reason is that it offers some very "radio-quiet" environments: areas with a very low level of man-made radio signals. The radio-wave receivers on the SKA will have to be very sensitive to measure the faint radio signals from the distant cosmos, and a radio-quiet environment will enhance the SKA's ability to detect these signals.
The SKA should preferably be situated within about thirty degrees of the Equator so that it can view the largest possible volume of sky. A Southern Hemisphere location would let astronomers see the centre of our own Galaxy, which passes overhead in the Southern Hemisphere.
How would Australia benefit from hosting the SKA?
Australia is currently a world leader in the technology associated with radio astronomy and in the astrophysics of sources that emit radio waves. The development of the SKA in Australia will enable research engineers and scientists to continue to support Australian industry through innovation, design, training and technology transfer. The Australian industries involved in constructing the SKA will be at a distinct competitive advantage in the area of spin-offs into products and systems for the information technology and communications industries.
The SKA is an international project. Australian companies that participate in such a major international project have the opportunity to demonstrate their capabilities internationally, develop opportunities for expansion of activities in Australia and overseas, and widen the base from which they can draw expertise for future projects and developments.
There are social, industrial and financial benefits for the communities hosting the SKA, including:
- employment opportunities from provision of infrastructure works, ongoing engineering and support services, and increased tourism;
- better service infrastructure (e.g. roads); and
- increased opportunities for expanding local education and training in science and technology, and increased likelihood of attracting top scientists to the State where the SKA is sited.