The Big Bang & the Standard Model of the Universe
What is the Big Bang?
The "Big Bang" is the term given to what is currently the most widely accepted scientific model for the origin and evolution of the Universe. This model has supplanted other models such as the Steady State theory proposed by Hoyle, Bondi and Gold in the 1940s. Indeed it was Fred Hoyle who coined the term "big bang" as a derisory one in an interview in the 1960s.
In the Big Bang theory the Universe comes into existence, creating time and space. Initially the Universe would have been extremely hot and dense. It expanded and cooled. Some of the energy involved was turned into matter. Current observations suggest an age for the Universe of about 13.7 billion years.
The current success of the big bang model relies on several key areas of observational evidence and predictions. These are discussed briefly below.
Evidence for the Big Bang Model
There are several key areas of observational support for the big bang model. These are:
- Observed recession of galaxies: The consensus among astronomers is that Hubble's relationship between the distance to galaxies and their recession velocity is due to the expansion of space. More distant galaxies or clusters of galaxies exhibit higher redshift of their spectral lines than closer galaxies. This is then interpreted as more distant galaxies receding from us faster than closer ones. Note it is important to realise that it is the space between galaxies that is expanding. Galaxies themselves do not appear to expand as the local effects of gravity dominate over any space expansion.
- Cosmic Microwave Background Radiation: In 1965 two scientists working for Bell Telephone Laboratories, Arno Penzias and Robert Wilson were adapting a horn-shaped antenna near New York for use in radio astronomy. They encountered noise in the system and despite repeated and thorough attempts were unable to remove it or find its cause. They eventually realised that this "noise" was in fact remnant radiation from the big bang. Such radiation had been predicted by Gamow in the late 1940s. As the Universe expanded it cooled so that today the background radiation corresponds to a temperature of 2.725 K and has a black body spectrum.
Credit: NASA, WMAPThis plot shows the black-body nature of the cosmic microwave background radiation. The spectrum corresponds to background radiation with a temperature of 2.725 K. These measurements were made by the FIRAS instrument on the COBE satellite. The error bars for each measurement are smaller than the width of the red line.Over the last 15 years observations of this cosmic microwave background radiation (CMBR) from space-based missions such as COBE and WMAP and balloon-based missions such as BOOMERanG that operated in the Antarctic have provided a wealth of details. We can now view the slight fluctuations or anisotropies in the CMBR with unprecedented detail and compare observations with theory more thoroughly. The image below shows how the resolution of the CMBR has improved since its discovery in the 1960s. These slight fluctuations in the CMBR intensity are thought to provide information about slight variations in density in the early Universe.
Credit: NASA, WMAPComparison of the level of detail of fluctuations in the CMBR from the 1960s through to the current WMAP mission. - Ratios of primordial elements. Astronomers are able to measure the relative amounts of the light nuclei hydrogen, deuterium (an isotope of hydrogen with one proton and one neutron), helium-3, helium-4 and lithium-7 in distant, unmixed clouds of primordial gas. The relative abundances of these nuclei correspond with the calculated predicted ratios from the Big Bang model.
- Observed evolution of extragalactic objects over cosmic time. Evidence for this initially came from radio surveys which showed that the more distant (hence older) parts of the Universe appeared to contain stronger radio sources than the local region. Quasars, for instance, are not found in our local region but are far more common at redshifts of 2 or 3.
Recent observations by the Hubble Space Telescope and other telescopes have provided our deepest ever views of the Universe and clearly show evidence of galactic evolution and earlier stages in their formation.
Formation of Matter
All matter, including the atoms in our bodies, the air we breathe and the gas in the Sun is composed is combinations of fundamental particles that were created during the Big Bang and subsequent evolution of the Universe. Before giving an outline of the key stages in the formation of matter we need to review the fundamental particles and forces in the Universe.
Fundamental particles, the building blocks of the Universe
Our current understanding of physics allows us to model events in the Universe nearly, but not quite back to the moment of the big bang. Significant developments in our understanding of the very early Universe are due to advances in high-energy particle physics and particle accelerators such as those at CERN. According to the "Standard Model" of particle physics we now know that all the matter around us is composed of combinations of only a few fundamental particles. These twelve particles fall into two families, quarks and leptons.
Quarks are the particles that group together to form hadrons. Hadrons made of three quarks in turn are called baryons. The most familiar baryons to us are the protons and neutrons that comprise the nuclei of the atoms in our bodies and the rest of the Universe. A proton comprises two up quarks and one down quark, whilst a neutron has two down quarks and only one up quark. If you study the following table you will see that quarks have charges that are fractions of the charge of an electron, e. Hence the overall or net charge of a proton = 2 × (+2e/3) - 1 ×(-1e/3) = +1e and the overall charge of the neutron is 0.
Leptons include three charged particles, the electron, muon and tau particle. Each of these has an associated neutrino particle that is neutral.
Together these twelve particles are the building blocks of matter. Interestingly though, each of them has a corresponding antiparticle. These differ only in having the opposite charge but have the same mass as the corresponding matter particle. These antiparticles collectively are known as antimatter.
Family | Name | Charge | Rest Mass | Symbol |
---|---|---|---|---|
Quarks | up |
+2e/3
|
~ 4 MeV |
u
|
charm | ~ 1,250 MeV |
c
|
||
top | ~1,784,000 MeV |
t
|
||
down |
-1e/3
|
~ 6 MeV |
d
|
|
strange | ~ 110 MeV |
s
|
||
bottom | ~ 4,100 MeV |
b
|
||
Leptons | electron |
-e
|
0.511 MeV |
e-
|
muon | 105.7 MeV |
μ-
|
||
tau | 1784 MeV |
τ-
|
||
electron neutrino |
0
|
<2.5 eV |
νe
|
|
muon neutrino | <170 keV |
νμ
|
||
tau neutrino | <18 MeV |
ντ
|
Quarks can be grouped into three families, the up & down, the charm & strange and the top and bottom quarks. Each successive family has more massive particles. This trend is also followed by leptons; the electron and its neutrino are lighter than the muon and muon neutrino which in turn is lighter than the tau and tau neutrino.
Fundamental Forces
All interactions within the Universe arise due to only one of four fundamental forces:
Force | Example | Relative Strength (at 10-15m) |
Range | Exchange Particle |
---|---|---|---|---|
Gravitational | Acts on all objects with mass. Responsible for orbit of Earth around Sun. Binds stars, planets and galaxies together. |
10-38
|
Long range (infinite) | Described by general relativity as due to curvature of space-time. Graviton is hypothesised exchange particle |
Weak | Involved in transmutation of a neutron to a proton in Β- decay. |
10-13
|
<10-18m | W+, W- and Z0 bosons |
Electromagnetic | Interactions between charged particles. Responsible for light and chemical properties of matter and |
10-2
|
Long range (theoretically infinite but limited due to canceling effects of + and - charges and magnetic poles) | photon |
Strong | Binds nucleons (protons and neutrons) together in nucleus |
1
|
distance of adjacent nucleons in nucleus (10-15m) |
gluons |
Immediately following the Big Bang all four forces are thought to have been combined and governed by one one Theory of Everything. As the Universe expanded the energy density dropped and the forces started to split from each other. The first split occurred when gravity separated from the others at the Planck time, about 10-43 s. The Universe was at a temperature of 1032 K at this stage. Electromagnetism and the strong and weak nuclear forces were still combined as a Grand Unified force.
At 10-35 s and a temperature of 1027 K the strong nuclear force separated from the electro-weak force. These two then separated at about 10-12 s when the Universe was at 1015 K to give rise to the four distinct forces in our Universe today. Whilst gravity is the weakest of all these forces it now governs the evolution of the Universe due to its long range influence and the amount of matter present.
Particle Production and Nucleosynthesis
The intense energy released in the Big Bang provided the source of all the matter in the Universe. Quantum physics explains the production of particle-antiparticle pairs. Whilst most of these went on to mutually annihilate each other, producing gamma photons, a very slight imbalance of matter over antimatter provided the building blocks for nuclei and atoms.
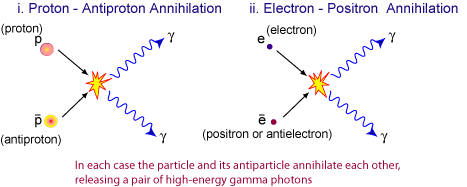
Within three minutes of the Big Bang, fusion reactions between protons and neutrons had made helium and deuterium nuclei. This process is called nucleosynthesis. 370,000 years later the Universe had expanded and cooled enough that the electrons could form stable atoms of hydrogen and helium. At this point matter is said to decouple from radiation and the Universe became transparent to photons. Light could thus travel long distances. We can view this event as the CMBR. The diagram below shows one of the possible nucleosynthesis sequences responsible for the production of helium-4 nuclei from protons and neutrons. About 24% of the baryonic mass of the early Universe was helium, the other 76% hydrogen.
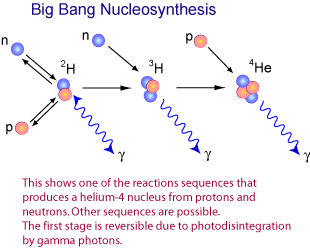
The table below provides an outline of the key events in the early history of the Universe.
Time since Big Bang | Temperature K |
Era | Key Events |
---|---|---|---|
0 |
∞
|
Radiation- dominated |
Big Bang. Universe formed. Time before 10-43s is termed the Planck time. Our Physics can not yet describe this interval in detail. |
10-43 s |
1032
|
Gravitational force separates from the strong-electro-weak force (Grand Unified force). Microscopic black holes form & disintegrate. | |
10-35 s |
1027
|
Grand unification ends (the strong force separates from the electro-weak force). Quarks, leptons and antiparticles created. Inflation occurs? Universe expands by factor of 1025. Gravitons form and decouple. |
|
10-12 s |
1015
|
Four fundamental forces now distinct. Leptons separate into electrons, neutrinos and antiparticles. Gravity starts to control expansion. |
|
10-6 s |
1013
|
Quarks & anti quarks form protons, neutrons & antiparticles. Protons & antiprotons; neutrons & antineutrons annihilate each other leaving slight excess of protons & neutrons plus lots of photons. |
|
1 s |
1010
|
Neutrinos and antineutrinos decouple. | |
15 s |
3 × 109
|
Electrons and positrons annihilate each other. Slight excess of electrons left (= number of protons so net charge of Universe is 0) plus more photons. | |
3 minutes |
109
|
Protons and neutrons fuse into helium nuclei (nucleosynthesis). Spare neutrons used up. | |
370,000 years |
3,000
|
Matter- dominated |
Matter decouples from radiation - electrons orbit nuclei to form atoms. Universe becomes transparent to photons as they can now travel long distances without interacting with charged particles. This decoupling is now viewed as the 2.7 K Cosmic Microwave Background Radiation. |
1 billion years | First stars and galaxies form. Heavy elements created in supernova explosions. | ||
8.4 billion years | Sun and solar system formed. | ||
13.4 billion years (NOW) |
3
|
Humans on Earth |
The Big Bang model successfully accounts for the formation of the light elements, hydrogen, helium and traces of lithium and their isotopes from fundamental particles. Elements heavier than helium, including the iron, carbon and oxygen in our bodies and the uranium in the Earth were all synthesised later in stars. Our bodies are made of the debris of earlier generations of stars.
Modern Cosmology
Our understanding of the Universe has undergone profound changes due to recent observations and discoveries. For some time astrophysicists have been aware that there is not enough visible matter in the Universe to account for the gravitational cohesion of clusters of galaxies or the rotation rate of spiral galaxies. These galaxies are spinning too fast for the observable matter in them to hold them together. To solve this dilemma the concept of dark matter has been suggested. This is matter that cannot be seen (hence dark) but otherwise interacts gravitationally with normal matter. A range of candidates from neutrinos, WIMPS (weakly-interacting massive particles) and MACHOS (massive astrophysical compact halo objects) have been proposed. Whilst some have now been ruled out there is still no consensus as to what dark matter is. The search continues.
Perhaps even more intriguing than the search for dark matter is the discovery, based on observations of distant supernovae, that the Universe is not just expanding but actually accelerating. Astrophysicists have proposed the concept of dark energy in the Universe. This acts as a repulsive force over the large scale, overcoming gravity. As with dark matter we do not yet know what this energy is in any detail.
These two discoveries combine to give us a very different picture of the Universe from that of a few decades ago. We now believe that all the "ordinary" matter that us, stars and galaxies are made of comprises only a small fraction of the constituents of the Universe.
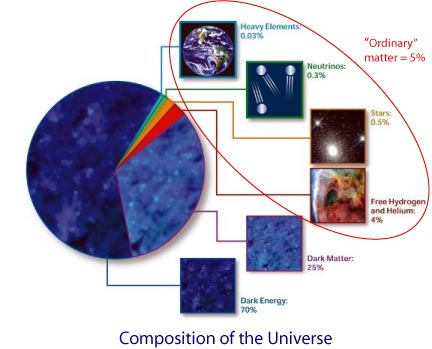
This section has provided only a brief introduction to the Big Bang. There are still problems to be solved and questions to be tackled but the Big Bang modelremains our best model for understanding the origin and evolution of the Universe at present. If you want to read more please go to many of the excellent external sources listed in our links page.